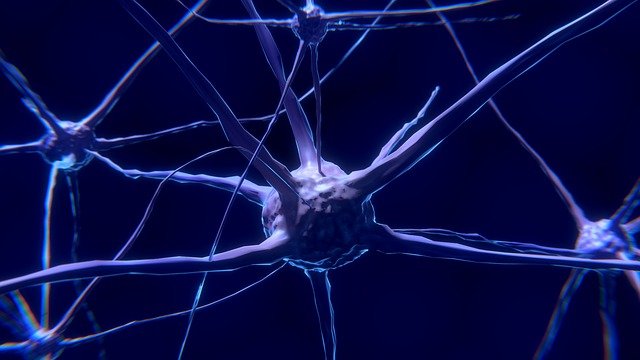
Neurodegenerative disease is a growing issue throughout the world. It is a collective term for a group of conditions that affect the neurones of the brain. The affect is the loss of function in the brain because of destruction of these neurones.
The diseases are irreversible and progressive.
The underlying pathophysiology has a few common issues. These are neuroinflammation which involves oxidative stress of the neurones accompanied by mitochondrial dysfunction.
The three most common diseases in this group of neurodegenerative diseases include Parkinson’s disease, Alzheimer’s disease, Huntingdon’s disorders and ALS. Thedr is enough research now showing that each condition is linked to oxidative stress and mitochondrial dysfunction.
Here we discuss some of the underlying biochemical mechanisms of oxidative stress which is a major cause of neuronal damage as it is with a number of cells throughout the body. We also examine in summary the role that phytochemicals might play in various aspects of minimising the impact of oxidative stress. This is a way of relating the healthy food we eat to why we can stem the tide of loss of brain function. We can never say phytochemicals are a cure but there are increasingly stronger associations to support the view that some of the compounds we ingest can help reduce these difficult neuronal degenerative diseases.
The Brain Is Peculiarly Susceptible To Oxidative Stress
The brain is an organ which is one of the most metabolically active in the body. It is remarkably prone to damage from oxidative stress for the three following reasons:
(1) There is a high demand for oxygen to keep it functioning properly. Although the brain is only 2 per cent by weight of the body, it consumes something of the order of 20 per cent of the total body oxygen to meet its metabolic demand.
(2) Compared to other organs, the brain has a lower antioxidant capacity.
(3) The brain contains a substantial amount of polyunsaturated fatty acids (PUFAs). These are prone to oxidation.
Basic Oxygen Chemistry
Molecular oxygen O2 is a biradical. The outermost shell of each oxygen atom in the molecule has a single electron. These two odd electrons occupy different orbitals and have the same spin. The brain is rich in oxygen and fuel provided by PUFAs. With so much oxygen it is surprising the brain does not autocombust but oxygen is prevented in this reaction because of the spin and orbital restriction within oxygen.
Reactive oxygen species (ROS)
A single electron reduction of oxygen generates the infamous superoxide anion. This is now a true free radical because it has now an odd free electron. It can be converted to hydrogen peroxide by addition of a hydrogen atom. This molecule is capable of oxidising a range of biomolecules and in turn disrupting cell membranes.
With transition metals such as iron, hydrogen peroxide, forms via the Fenton reaction, the highly reactive hydroxyl radical (•OH) which is converted to water by addition of a hydrogen atom in the form of an electron and a proton.
Molecular oxygen is converted to singlet oxygen by the promotion of the single electron in one of the outer shells to pair with the electron in the adjacent oxygen shell. Singlet oxygen is also a potent free radical.
In turn all the reactive oxygen species (ROS) collectively include these three species, the superoxide anion, the hydroxyl radical and singlet oxygen.
Nitric oxide is a compound formed endogenously within cells. It too is highly reactive. If nitric oxide is added to the superoxide anion, then a compound called peroxynitrite is generated (•ONOO). This is a potent oxidant and can also be added to the collection of reactive oxygen species.
All these reactive species can attack all sorts of biomolecules. The main ones are described here. So ROS can damage lipids through peroxidation reactions to produce toxic aldehydes which we’ve often discussed before, proteins can be oxidised which leads to their cross-linking, oxidise DNA bases so damaging them, induce expression of a collection of proinflammatory genes which leads to the manufacture of inflammatory marker molecules including Nuclear Factor Kappa B (NFkB). The ROS will also react with carbohydrates to form advanced glycated endproducts (AGE) which implies destruction of various key sugar molecules. They also cause mitochondrial damage. In summary, ROSs are highly damaging through their oxidative ability.
Avoiding Oxidative Damage
Cells have set up endogenous defense systems to minimise oxidative damage which are based around antioxidants. When the environment atmosphere became oxygen rich, the evolutionary defence mechanisms that developed included a set of enzymes to ameliorate damage from oxygen. The enzymes were:
- superoxide dismutase (SOD) (converts superoxide radicals to less reactive species)
- catalase (catalyses conversion of hydrogen peroxide in the liver to water and oxygen)
- glutathione peroxidase (also neutralises hydrogen peroxide)
- proteins such as ceruloplasmin, ferritin which sequester metal ions and prevent them from taking part in the Fenton reaction.
In general, there is a balance between oxidative stress and antioxidant defense. Preferably, the balance should be in favour of antioxidant defense. If it is tipped in favour of oxidative stress then organ damage ensues and the brain is especially affected.
Mitochondrial Dysfunction In Neurodegenerative Disease
Mitochondrial dysfunction can lead to apoptosis which is the most common form of programmed cell death in neurodegeneration. ROS are byproducts of normal mitochondrial respiratory chain activity.
Mitochondria are dynamic organelles and the powerhouse of the cell but they can also turn into creators of poisonous molecules which in turn damage themselves and the surrounding organelles of the cell.
The mitochondria has an outer membrane with a series of inner membranes in the form of lamellae. On the surface of the inner membranes resides the electron transfer chain which traverses this membrane.
The electron transport chain or complex of the mitochondria has five different sub units denoted I, II, III, IV and V. In normal mitochondrial function the electron transport chain converts three molecules of oxygen to three of singlet oxygen, and a molecule of ATP is formed from ADP. The ROS are produced by complex I and III. These ROS are released into the mitochondrial matrix and the intermembrane space as a result of this normal reaction. Under normal circumstances of metabolism, these are neutralised by superoxide dismutase, catalase and glutathione peroxidase using glutathione as the radical receiver in the latter case.
If the mitochondria is damaged then the electron transfer chain starts to malfunction, where complex I and III end up producing an over abundance of ROS. These are not mopped up and start to damage the mitochondria and surrounding cell structures.
The mitochondria has an outer and inner mitochondrial membrane. The outer mitochondrial membrane has a voltage-dependent anion channel (VDAC) traversing it. The inner mitochondrial membrane has a complementary protein gate known as the adenine nucleotide translocator (ANT). The ANT transports an ATP molecule out of the cell in exchange for and ADP which comes in. In the mitochondrial matrix there are additional proteins called cyclophilin D.
In response to oxidative stress, a mitochondrial permeability transition pore (mPTP) is created between the VDAC and ANT.
This is generated by cyclophilin D which attaches itself to the adenine nucleotide translocator (ANT). Once this attachment is created, the ANT aligns with the voltage-dependent anion channel (VDAC). The alignment opens up a channel also called a pore which is the mitochondrial permeability transition pore (mPTP) between the mitochondrial cytoplasm and the external environment of the mitochondria.
The pore (mPTP) allows the escape of mitochondrial contents into the cytoplasm of the cell. It is mainly cytochrome c. This pore is called the ‘gate of death’. It is one of two ‘gates of death’.
The second gate of death is the MOMP which is a gated protein complex in the outer mitochondrial membrane known as the Mitochondrial Outer Membrane Permeabilization (MOMP). This gate is regulated by proteins called the BCL-2 like proteins and include Bax and Bak. These two proteins are present in the mitochondrial membrane and when activated bind to the MOMP gate which opens up the channel. This channel also releases cytochrome c.
The outer mitochondrial membrane also has two other proteins called Bcl-2 and Bcl-xl which inhibit the actions of Bax and Bak. When Bcl-2 is stimulated it results in the closure of the channel (pore) and stops the escape of cytochrome c. There are phytochemicals which also block Bax and Bak.
The cytochrome c which is released by the mitochondria activates caspases such as 3, 6 and 7. These are cysteine proteases which can cleave DNA and trigger neuronal apoptosis. This is part of the programmed cell death mechanism. A leak of cytochrome c is the agent that promotes programmed cell death of neuronal cells.
Mitochondrial Biogenesis
Mitochondrial biogenesis may have direct influence in the process of neuronal degeneration.
Damage to a neuronal cell leads to upregulation of an enzyme called SIRT1. SIRT 1 catalyses the deacylation of PGC1α for example. This particular molecule enters the nucleus and binds to Nrf1. The complex of PGC1α and Nrf1 upregulates the gene Tfam coding for the product Transcription Factor A. This TF-A enters the mitochondria of the neurone and upregulates the mitochondrial DNA. This ultimately leads to production of more mitochondria.
Mitochondrial biogenesis means improved mitochondrial function and with that better survival rates for the neurone.
Phytochemicals such as resveratrol can also stimulate SIRT 1 and also cause neuronal survival.
Antioxidant Protection Against Neurodegenerative Disease Is provided By Phytochemicals
Food offers some potential sources of agents that could help to ameliorate the damge of oxidative stress in the body and in particular reduce the development of neurodegenerative disease.
A number of phytochemicals act as free radical scavengers of both ROS and RNS (reactive nitrogen species). They include molecules such as curcumin, the flavonoids, such as apigenenin, kaempferol and leuteolin.
In the neurone nucleus, iron which is involved in the Fenton reaction is chelated by a host of flavonoids such as baicalein, myricetin, quercetin and EGCG. There are also non-flavonoids involved too such as gallic acid and protocatechuic acid.
Some flavonoids also inhibit the activation of proinflammatory genes. These include flavonoids, lycopene, curcumin and stilbenoids.
Some flavonoids can also protect directly against mitochondrial damage by raising the activity of the electron transport chain in the mitochondria. There is evidence for this using EGCG, hesperidin and quercetin.
A similar group of phytochemicals can also improve mitochondrial biogenesis. These include EGCG, resveratrol, quercetin, genistein and hydroxytyrosol which comes mainly from olives.
The gates of death can also be prevented by forming so that cytochrome c does not leak into the cytoplasm. Resveratrol and rosemarinic acid have been shown to do this.
Finally, neuronal apoptosis too is inhibited by inducing the expression of the proteins such as Bcl-2 and Bcl-xl or by suppressing the behaviour of Bax and Bak. The phytochemicals quercetin, astaxanthin and naringenin are associated with this activity.
The main effort of nutritional research in reducing neurodegenerative disease is to achieve a miracle cure to block neuronal apoptosis. Phytochemicals appear feasible in this endeavour by ameliorating neuronal degeneration. The agents need to be site-specific especially if they can be directed at those sites where the free radicals are created in the neurones. The antioxidant also has to have a higher rate constant that those of natural biomolecules when it comes to mopping up free radicals. It must compete with these natural biomolecules in that process.
One issue is the poor solubility of some of these phytochemicals. Curcumin is not very soluble at all and is rapidly degraded in the gut before it even gets to the site of action. There is also the role of homeostasis because a redox balance operates in the body to regulate the levels of prooxidants and antioxidants.
Further information on the subject is obtainable from the web-site on neurodegenerative research.
Leave a Reply