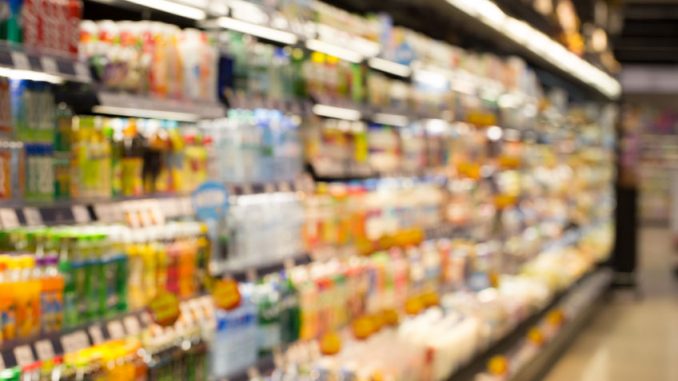
Of all the emerging technologies to have entered the field of food processing in a major way, high pressure processing (HPP) is perhaps the one that has had the greatest impact. The main attractiveness of the technology is that it doesn’t need heat so the food that is processed is stabilised long enough without sacrificing any of the fresh characteristics. It is now the most popular of all the non-thermal processing technologies.
HPP Satisfies A Variety Of Attributes
Freshness has always been a major demand of consumers for any food and whilst high pressure processing has caveats, it more or less satisfies this demand.
The other key labels are maintained levels of food safety, preservation of a food’s nutrition, part of the clean label idea, is still being innovated upon and sustainable
HPP As A Processing Method
High pressure processing (HPP) encompasses two forms:
- static or high hydrostatic processing (HPP)
- dynamic or high pressure homogenisation, (HPH)
In this article we will only look at HPP because it has become so universal as a technique. HPH is worth considering in its own right and we will cover in another article on this subject.
The main purpose of applying the technology is to inactivate microorganisms and enzymes which can seriously damage a fresh food. The main advantage over other stabilising technologies is that it is non-thermal. The processing temperature to all intents and purposes never rises above 40 C. What makes it even more attractive is its suitability for processing heat sensitive products such as avocado as in guacamole, fine hams and fresh fish. Processors actually use high temperature with high pressure to thoroughly treat products to the point where they are sterilized (Wilson et al., 2008).
Having stated that it is only relatively recently that HPP has come to the fore, Hite in 1899 was discussing the use of high pressure to pasteurise milk when he was working at the West Virginia University’s Agricultural Experiment Station. He also reported on the effect of HPP on the microbial load in fruits and vegetables (Hite et al., 1914).
The technology was placed on hold as it were for nearly 50 years until the Japanese began to examine the process for jam making. The first commercial units started in Japan for the pasteurisation of refrigerated acidic foods. Such foods were marketed in Japan in 1990. Europe and the USA began to advertise HPP treated brands in 1996 and then Australia in 2001. Commercialisation seriously took off from the 2000s.
The principles and methods for high pressure processing are well covered in the literature. Please look at the review by Farkas and Hoover (2000) for a general overview. A more up to date version is by Hogan et al., (2005), Torres and Velazquez (2005), Muntean et al., (2016).
The Principles Of High Pressure Processing
Pressure causes all sorts of chemical and physical changes when it is applied. As in Boyle’s Law, when pressure is applied there is a physical compression with a reduction in volume and an increase in temperature and energy (Heremans, 2003). The physical changes in pressure and volume are encapsulated in the Le Chatelier- Braun Principle.
In HPP, high intense pressures of between 400 and 600 MPa (58,000 to 87,000 psi) are applied to products which are chilled or submitted to processing at ambient temperatures at least below 45 Centigrade. HPP is applied in a batch process to liquid to solid or gel-like high-moisture content foods. You can find applications that will reach the heights of 900 MPa although there is little benefit in going beyond 600 MPa in most cases.
Benefits Of High Pressure Processing
The low processing temperatures mean colour, aroma and preservation of nutritional factors are retained. It also means the organoleptic and rheological factors are generally preserved.
One of the benefits of HPP is a food processing and engineering one, where the size and geometry of the sample during processing does not matter. It can also operate in as a waste-free and environment-friendly piece of technology. For many this represents a breakthrough in gentle processing (Toepfl et al., 2006).
Generally, HPP does not break covalent bonds. The effects are felt at higher molecular weight levels because it makes structures become more ‘ordered’. That means their three-dimensional structure alters by changing hydrogen bonds. The types of molecules disrupted by changes in their secondary and tertiary structure are carbohydrates and proteins. There is a complete change in their structural and functional integrity. The microorganisms are killed because of the extreme damage to their cell membranes and any membranes surrounding their organelles. However all the affected microorganisms are prokaryotes so the impact is not so great (Kalchayanand et al., 1994; 1998).
The Disadvantages Of HPP
The limitations of HHP are mainly the overall expense of the equipment. Foods need to have 40% free water for its anti-microbial effect. It is still a batch process even though semi-continuous and pulsed processing has been tried. There is still some microbial survival which means suitable HACCP must be in place to ensure quality and no pathogens evade processing. If food is processed at ambient temperature, very few bacterial spores are inactivated (Heinz & Knorr, 2002). For a good or bad, there is little effect on food enzyme activity.
Whilst HPP is not meant to affect pigments in food this is not entirely accurate. Myoglobin in meats and tuna can be blanched (Cheftel & Culioli, 1997) and there seems to be a process of oxidative degradation occurring to pigments like astaxanthin in salmon flesh which is coupled to a related globin pigment, metmyoglobin (Tintchev et al., 2009, 2010).
HPP also causes temperature increases as well as pressure increases. It is sometimes a neglected phenomenon because of the marketing adherence to ‘cold processing’ as a concept. There is thought to be a rise in temperature of the liquid component of a food of roughly 3°C per 100 MPa increase. When the fat content rises, the temperature rise is between 8 and 9°C (Rasanayagam et al., 2003).
HPP does not effectively deal with spore-formers such as fungi.
The Effect Of High Pressure On Microbes
The FDA defined four types of inactivation which are based on the following:-
- cellular changes induced by a rapid change in pressure
- changes in biochemical mechanisms
- changes to the porosity of cell membranes
- alteration to the mechanisms regulating genes and gene structure
Cell morphology alters with applied pressures between 100 and 300 MPa, slowing down cellular division. Enzyme activity is disrupted because of the changes to the inter- and intramolecular structure which produces conformational change at the active site. The cell membrane is damaged because variable mechanisms to control cell volume are disrupted. The FDA claim damage is equivalent to the application of high temperatures and oxidative stress.
Microorganisms Killed By HPP
The critical stabilising feature of HPP is its ability to inactivate if not kill pathogenic and spoilage microorganisms. Given that spore inactivation was not feasible in early uses of the equipment, there is now a concerted effort to examine medium to high temperatures as a means of inactivating bacterial spores. To be able to understand what conditions of HPP to apply in terms of pressure, time and temperature, it is necessary to know how the microorganisms are inactivated. Knowledge of their cell walls, membranes, genetic mechanisms and key enzymes should all be taken into account as well as the mechanisms that allow sublethal recovery.
The food safety microorganisms of any note are Listeria innocua, various Clostridium spp. such as C. botulinum, C. difficile and C. perfringens, Salmonella, Vibrio spp. such as V. parahaemolyticus and V. vulnificus, Staphylococcus and many others.
A good review on this aspect is covered by Mor-Mur et al., (2014).
Pressure-assisted thermal sterlization (PATS) which uses temperatures of 500 to 900 MPa combined with thermal treatment between 90 and 120 °C is probably the only effective method for inactivating spores (Koutchma, 2014).
The FDA in the USA requires that a process generating a shelf-stable juice for example should demonstrate at least a log 5 reduction in the microorganism population. In tiger nut milk which is an example of a non-dairy milk, a 6-log reduction in E. coli, Salmonella spp. and L. monocytogenes is possible (Elberhami et al., 2016). Low-acid foods are susceptible to inadequate processing of all sorts because a pH below 4.5 is a specific hurdle whilst above there is significant risk from the growth of bacteria like Bacillus cereus and Clostridium botulinum.
The pressures required to achieve a 5-log reduction in one cycle over a 15 minute period has been calculated. The most resistant is Staphylococcus aureus which needs 700 MPa, then E. coli O157:H7 (680 MPa), Salmonella enteritidis (450 MPa), Listeria monocytogens (375 MPa), Salmonella typhimurium (350 MPa) and finally Yersinia enterolcolitica (275 MPa).
The Effect Of HPP On Reducing The Risk Of Fungal Spoilage.
One aspect of microbial spoilage which is an issue for fruit juice producers in particular is destroying spore-formers especially those of fungi. The types of fungi found in fruit juices in particular include Penicillium spp., Aspergillus niger, Byssochlamys spectabilis, Rhodotorula mucilaginosa, Candida parapsilosis, Torulaspora delbrueckii, or Brettanomyces bruxellensis.
One early study on the survival of spoilage fungi using HPP looked at pressure sensitive fungi. The researchers compared HPP and thermal pasteurization on the shelf-life of guava pureé (Yen and Lin, 1996). These pureés which were processed at 400–600 MPa for 15 minutes at 25 °C or 88–90 °C for 24 s, along with an unprocessed control, were stored for 2 months at 4 °C. The puree treated with the highest pressure at 600 MPa was able to initially reduce the naturally occurring yeasts and molds to what is described as non-detectable levels. When they did thermal pasteurization or a lower pressure processing such as 400MPa, the fungal population was reduced by only one log count. During the 4 °C shelf life study, those samples processed at 600 MPa did not show recovery of cells, but the control, 400 MPa, and thermally pasteurized samples showed growth after the first 10 days.
One very good study looked at the effect of water activity (aw) and pH on the survival of fungi in an apple juice treated with HPP (Buerman et al., 2020).
in this study, the fungi recovered from the HPP treatment and continued to spoil the juice by their subsequent fermentation. The factors that were found to reduce the spoilage to a certain extent was to raise the level of water activity and make the pH more acidic. This is in keeping with HACCP principles generally.
Of all the fungi, the Candida species were the most pressure resistant.
Equipment For HPP processing
The equipment for HPP treatment of any food is the following:-
- A pressure vessel in a cylindrical design
- Two end closures
- A means to restrain the end closures such as a yoke, threads or a pin
- A low pressure pump
- An intensifier using liquid fro the low pressure pump to generate the high pressure process fluid for system compression
- Significant system and process control
There are six components which can be arranged into a semi-continuous operation for unpackaged liquid foods or for packaged foods in a batch operation.
Manufacturers Of HPP Equipment
The technology has been expensive to run because of its batch nature however there the technology is becoming a more semi-continuous process. It is more commercially viable the more costly the food to treat.
Typical producers include:
- Alstom in Nantes, France.
- Avure (Erlanger, Kentucky, USA)
- Hiperbaric (Miami, Florida, USA: Spain)
- Thyssen-Krupp
Coldpressure.org is the HPP trade organization which has plenty of advice to offer on the process.
Commercial Operation Of A HPP Unit
A typical piece of equipment might be a 215 litre Flow International pressure vessel with appropriate pressure pumps to generate an operating maximum hydrostatic pressure of 690 MPa (100,000 psi). The unit should be able to take a maximum temperature of 100 C with a 90 second minimum pressurization time and a 30 second minimum depressurisation time. A cycle time might typically be between 6 to 8 minutes. Typically, these times are all relatively longer. Back in 2010 the cost would have been $3.5 million but inflation may well see costs have risen to $5 million.
A conventional operation might have five of these units which means that approximately 390,000 pounds of food in processed every day. If it operate for 330 days in a year, there is a 90% up time than the total annual output is close to 135 mill. Lb/year. This is assuming a product is prepackaged with a 75% load rate. The depreciation on such a piece of kit has to be assumed but for argument’s sake it can be just 10 years.
The capital cost for 5 HPP units totals $17.5 million. Housing them in a specific building with all the support materials could eb $10.5 million which makes a total of $28 million.
On the basis that costing a unit process in pounds of food, the labour costs is $0.009/lb, the maintenance is 0.018/lb, the depreciation is $0.0185/lb then he total costs of processing a pound of food in this sum of 45.5 cents per lb.
A typical process for sausages would be to treat at 300MPa for 10 minutes with the temperature recording at maximum, 17 C. the come up time to pressure would be about 9 minutes with a pressure release taking 1.5 minutes.
What Next?
The goal of a continuous HPP process is the next step. No commercial continuous HPP system exists. A continuous system must compress the liquid food with it operating in a plug flow holding tube or holding vessel for a specific process time. The liquid must then be able to decompress so that the liquid is able to do work which minimises if not avoids altogether excessive shear and heating. The decompressed treated liquid is probably sent to a sterile hold tank for eventual aseptic filling.
It is also feasible to combine non-thermal treatments. One good example combined HPP with pulsed electric field processing and sonication (Pyatkovskyy et al., 2018). One good example showed that a sequential; HPP followed by PEF treatment was additive in its lethal effect on Listeria innocua in water. If HPP and PEF were simultaneous there were synergistic effects. They noted an increase in the suspension’s zeta potential with the degree of Listeria inactivation. The leakage from the cells were mainly non-protein molecules.
Types of food processed
- Avocado puree and other vegetable pastes
- Chinese Rice Wine (Yang et al., 2019)
- Fruit juices, purees, pulps, nectars
- Non-dairy milks
- Chickpeas and other legumes
- Processed hams
- Fish pastes, whole fish
- Low-acid fermented sausages
- Minced beef (Carlez et al., 1993; 1994; 1995)
- Salads (Pandrangi et al., 2014)
- Ready meals (Pandrangi et al., 2014)
The only foods that are not processed are ones containing air. It is not suitable for bread, any dry solid foods containing pockets of air because the product would collapse and shrink under water pressure. HPP can make refrigerated soups last longer but it wont really work for the low-acid, shelf-stable types.
Types Of Packaging In High Pressure Processing
The packaging as well as the product to be treated is subjected to pressures between 400 and 900 MPa with water as the pressurization fluid. In that case all packaging must be water resistant no matter what because it comes into contact with pressurised water during the HPP cycle. Likewise it cannot leak !
The packaging must be flexible enough because during pressurization as the water volume reduces by 15 to 18% but returns when the HPP cycle ends. The packaging must accommodate this change both during compression and relaxation of pressure. Plastic has always been the packaging of choice because it has food flexibility, water barrier properties and a certain degree of elasticity when pressure is applied. Materials not suitable for HPP are paperboard-based, metal cans and glass bottles. Why ? They do not recover shape and size following HPP because they have little pressure and water resistance.
Most liquid foods are packaged into flexible plastic types such as PET or any other conventional packaging. Most solids foods are placed in plastic bags such as polyamide-polyethylene and then processed. Vacuum packed foods are probably the best suited to this type of processing.
As well as good mechanical, barrier and sealing properties, all HPP packaging needs to comply with international, national and regional regulations regarding food-contact materials and migration. All the food authorities in the USA, Europe and Canada provide the key guidelines and information on this aspect.
High Pressure Processing Of Fruit Juices
Fruit juices are susceptible to microbial spoilage and enzymatic activities and thus have a limited shelf life. High hydrostatic pressure (HHP) treatment can be used to inactivate microorganisms and enzymes. The development of reliable and safe HHP processes will require detailed knowledge about the HHP inactivation of pathogens with an adequate margin of safety, and how pressure–time–temperature combinations and the juice type affecting the inactivation (Cheftel, 1992; Smelt, 1998). The studies showed that different strains of a species can have widely varying pressure resistance and the stage of growth of bacteria is important in determining pressure resistance. Cells in stationary phase are more pressure resistant than those in the exponential phase (Benito et al.,1999; Isaacs & Chilton, 1995; Patterson, et al; 1995).
Although food poisoning outbreaks have often been associated with the consumption of foods of animal origin, outbreaks associated with traditionally consumed acid foods were also reported (Cody et al., 1999; McCarthy, 1996). As pressure resistance of bacteria varies among foods it is important to validate processing parameters in foods and not extrapolate results from buffers and laboratory media. Therefore, in developing pressurization parameters, pressure-resistant strains of bacterial species should be used as surrogates to ensure greater safety of foods. The effect of low temperature, high pressure treatment on the survival of mainly gram-negative foodborne pathogens, E. coli O157:H7 and Salmonella, inoculated in different fruit juices has been reported in literature (Linton, et al., 1999; Teo, et al., 2001. It has also been reported that gram-negative bacteria and cells in exponential growth phase, respectively, are more pressure-sensitive than gram-positive bacteria and early stationary phase cells (Hauben, et al, 1996; Kalchayanand, et al, 1998).
Based on the findings of the study on fungal spoilage organisms, it is better to process acidic pH juices which are more concentrated so that shelf-life is retained. That is in keeping with general processing principles for juices where generating a concentrate is much more effective for storage purposes.
High Pressure Processing Of Alcoholic Beverages
It should be no surprise that alcoholic beverages can also be processing using HPP. Chinese Rice Wine which is treated using HPP at between 400/600 MPa for 10 min produces a flavour which is virtually identical to the untreated product. What is missing though is a particular cereal aroma and astringency which heat treatment offers (Yang et al., 2019).
High Pressure Processing Of Non-Dairy Milks
There is very little work conducted on HPP processing of milks save for some studies on soy milk (Smith et al., 2009). A 3 log CFU per ml reduction using 600MPa is possible using HPP at 600 MPa which destroyed mesophilic bacteria in soy smoothies (Andrés et al., 2016a).
HPP helps to stabilise the proteins in soymilk whatever the temperature of processing. When the soymilk was heated to 75°C, most of the psychotrophic bacteria was destroyed. The process is so effective the soymilk was stabilised for 28 days with refrigerated storage compared to unprocessed product. The ideal processing conditions was pressure (600 MPa) at 75 °C for 1 min. because shelf-life was extended with improved protein stability. Most importantly, the bioactive compounds such as the isoflavones were not destroyed by HPP. Sensorially, HPP treated soymilk tastes virtually the same as untreated soymilk (Andrés et al., 2016).
The same author (Andrés et al., 2016b) also found HPP with thermal heating of a soy smoothies produced a cold storage product for 45 days using just 650 MPa treatment for 3 min at 20 °C. The length of stability was the same microbial safety as thermal pasteurisation (80 °C per 3 min).
A study on hazelnut milks has shown that HPP not only significantly reduced the levels of coliforms, yeasts, moulds, APC but that a 600 MPa treatment for 5 minutes was equivalent in pasteurisation efficacy as 80 °C (Tsai et al., 2018).
A Texas based business is producing MALK which are chill-chain nut milks (almond, pecan and cashew milk) in multi-serve 28oz and single-serve 12oz bottles (pecan & cold brew coffee; pure cashew and pecan & raw cacao). The producers claim a 49-day shelf-life. Clearly HPP can be a useful tool in preserving the flavour quality of these non-dairy milks if they have been successfully launched.
Product Changes: Enzymes
Applying HPP means that the foods are treated without heat processing and so minimally affecting the taste, appearance, texture and nutritional value of the product. The implication is that the small molecular weight compounds like vitamins, heat labile or unstable nutrients, pigments and colours, antioxidants, flavours and a host of other compounds all remain unchanged during and immediately after the process. It seems at least the changes are negligible enough not to affect the quality of the product. In the longer term there are changes that occur to all those quality attributes because residual enzymes remain active. Indeed some are activated such as polyphenol oxidase (PPO)!
One good example illustrating the effect of reactivation of enzymes such as PPO occurred in a study comparing high pressure processing with the thermal processing of strawberry pureé and juice (Aaby et al., 2018). This illustrated one of the main issues with HPP processing. Whilst the microbiological shelf-life of both pureé and juice was at least 49 days with HPP, on storage the losses of colour, anthocyanins and vitamin C in the pureé were substantially higher with this technique compared to a heat treatment at 85 °C for 2 minutes. The differences in storage quality of the juices using both techniques was not as significant. This study demonstrated an important point that with HPP treated purees, there was a higher retained enzymic activity. It also shows that juices are probably a better material than pureé for HPP processing. The other aspect is that heat processing does have some significant benefits which non-thermal processes have not yet been able to overcome.
To reduce the impact of endogenous enzyme activity and keep food quality as high as feasible means trying different approaches and procedures to minimise enzyme activity. One very comprehensive and well researched review on enzymes should be examined for its approach to understanding the mechanisms and kinetics of pressure induced degradation and denaturation of enzymes (Hendrickx et al., 1998).
So the following have been tried:-
- altering the pH usually by acidification
- refrigerated or chilled storage
- high-temperature treatment which seems to work against the concepts of HPP
- anti-browning agents
HPP not only alters enzyme activity it can have profound effects on the structure of the food by altering the matrix, affecting the cell integrity and improving water diffusivity. We know already that cell permeability is altered drastically because of the way it kills micro-organisms but it affects structural cells in plans and animal tissue too.
Product Changes: Gelling
HPP can markedly change the gelling properties of all sorts of proteins including β‑lactoglobulin, casein, soy protein isolate, surimi and mytolin amongst others. The process appears to change the protein structure either through denaturation and aggregation. The three-dimensional network is formed through protein folding which is stabilised by disulphide bonds, hydrophobic interactions, electrostatic interactions, hydrogen bonding and other types of amino-acid links.
Beta-lactoglobulin is a key globular protein in milk whey. Gelling can be induced using HPP (Li et al., 2018). When lactoglobulin gels are subjected to HPP the lowest protein concentration required to form β-Lg gels was 20% (w/v) at 400 MPa for 30 min at pH 3.0, 5.0 and 7.0, while 14% (w/v) protein was required to form gels at 600 MPa. Interestingly for food developers, gel strength and various textural properties increased as the protein concentration and the applied pressure rose. Gels produced at pH5 had the highest strength which may reflect on the isoelectric point for this protein. In this instance Raman spectroscopy showed that the α-helix content had dropped and the number of random coils had increase.
Rehydration
Dehydrated foods are always rehydrated before they are consumed. One of the issues sometimes with high pressure processing is the realization that solids have been lost during rehydration of a dried foodstuff. Rastogi et al., (2000) examined the changes that occur to both the moisture and solid contents of dehydrated pineapples following their rehydration. The pineapple pieces were treated using HPP to stabilise them before a two-stage drying process of osmotic dehydration and finish drying at 25 °C. They measured the diffusion coefficients for water infusion as well as solute diffusion. These were significantly lower in the high-pressure pre-treated samples. The dramatic drop in the water diffusion coefficient was attributed to the permeabilization of the cell membranes which reduced the rehydration capacity (Rastogi & Niranjan, 1998).
Studies on water adsorption and cooking time have also been examined with chickpeas. High-pressure treatment is claimed here not to have improved in terms of reduction in time (Ibarz et al., 2004).
High Pressure Processing And Processing Of Pulses (Beans And Peas)
Belmiro et al., (2018) examined the effect of high pressure processing on the hydration and drying curves of common beans (Phaseolus vulgaris L.). HPP improves processing of beans considerably. In this study the beans were processed in water in a HPP unit at 600 MPa. They looked at the effect on hydration, drying, rehydration, cooking time and final texture – so quite encompassing. The HPP process improved the hydration of the beans by up to 4.7 times faster. The water diffusivity was also 27% faster during drying whilst rehydration improved by 2.1 times after HPP. The cooking time was also reduced by 15 minutes because of the higher level of hydration and there were no negative effects on their final texture.
From a commercial perspective, HPP alters the bean’s structure sufficiently to improve their processing.
Use Of High Pressure Processing And Government Regulations
Currently the United States Food and Drug Administration (FDA), United States Department of Agriculture (USDA) and all international food safety agencies recognize HPP food technology. It is regulated as in any other food processing operation. There are no special labelling requirements and many brands like to let the consumer know that their products have been cold processed or cold pressed implying that HPP has been used. In many cases HPP is referenced as the process used in stabilising their product.
HACCP – The Critical Control Points
The product must be sterilized in which case a number of variables must be measured and recorded very similar to conventional retorting. The regulations include:-
Hazard Analysis and Critical Control Point Systems (Juice HACCP; 21 CFR 120)
Thermally Processed Low-Acid Foods Packaged in hermetically Sealed Containers (21 CFR 113)
Acidfied Foods (21 CFR 114)
Control of Listeria monocytogenes in Post-Lethality Exposed Ready-To-Eat products (9 CFR 430.4).
The Food Safety Modernization Act (FSMA) is more stringent and stipulates validation of HPP with the particular product under investigation. Specific pressures and holding times need to be defined and they are tied to a specific level of kill of the microorganism.
Research Groups Involved In High Pressure Processing
Queens University, Belfast has their AFBI group at Newforge.
Conclusions
Clearly, HPP offers the processor of foods all sorts of advantages especially when it comes to retaining quality but caution has to be given concerning the same properties. We know from studies on fruit purees that HPP does not destroy enzymes and these will happily degrade colour, vitamins, various nutrients and other factors which means long-term storage is not viable. In some cases it can even activate spoilage enzymes.
At the moment the technology is still finding its way in the commercial world. The main goal is to reduce the cost of processing because it is still a batch operation. Continuous processing is currently still a dream with HPP.
References
Aaby, K., Grimsbo, I.H., Hovda, M.B., Rode, T.M. (2018) Effect of high pressure and thermal processing on shelf life and quality of strawberry pureé and juice. Food Chem., 260 pp. 115-123 (Article)
Andrés, V., Villaneuva, M.-J., Tenorio, M.-D. (2016a) Influence of high pressure processing on microbial shelf life, sensory profile, soluble sugars, organic acids, and mineral content of milk- and soy-smoothies. LWT-Food Sci. Technol., 65 pp. 98-105 (Article)
2016b). High hydrostatic pressure treatment and storage of soy‐smoothies: colour, bioactive compounds and antioxidant capacity. LWT ‐ Food Science and Technology., 69, pp. 123–130 (Article) , , , & (
Bayndrl, A., Bozoglu, F., Alpas, H., Hzal, M. (2006) Efficiency of high pressure treatment on inactivation of pathogenic microorganisms and enzymes in apple, orange, apricot and sour cherry juices. Food Control, Vol. 17, (1) pp. 52-58
Belmiro, R.C., Tribst, A.A.L., Cristianini, M. (2018) Impact of high pressure processing in hydration and drying curves of common beans (Phaseolus vulgaris L.). Innov. Food Sci., Emerg. Technol. 47 pp. 279-285 (Article)
Benito,A., Ventoura, G., Casadei, M., Robinson, T. and Mackey, B. (1999) Variation in resistance of natural isolates of Escherichia coli O157 to high hydrostatic pressure, mild heat and other stresses, Applied and Environment Microbiology 65 (4), pp. 1564–1569
Buerman, E.C., Worobo, R.W., Padilla-Zakour, O.I. (2020) High pressure processing of spoilage fungi as affected by water activity in a diluted apple juice concentrate. Food Control 107 106779 (Article).
Carlez, A., Rosec, J.P., Richard, N., Cheftel, J.C. (1993) High pressure inactivation of Citrobacter freundii, Pseudomonas fluorescens, and Listeria innocua in inoc-ulated minced beef muscle. Lebensm Wiss Technol 26 pp. 357–63
Carlez, A., Rosec, J.P., Richard, N., Cheftel, JC. (1994) Bacterial growth during chilled storage of pressure-treated minced meat. Lebensm. Wiss. Technol. 27 pp. 48–54.
Carlez, A., Veciana-Nogues, T., Cheftel, J.C. (1995) Changes in color an myoglobin of minced beef meat due to high hydrostatic pressure processing. Lebensm. Wiss. Technol. 28 pp. 528–38
Cheftel, J.C. (1992) Effect of high hydrostatic pressure on food constituents: an overview. In: C. Balny, R. Hayashi, K. Heremans and P. Masson, Editors, High Pressure and Biotechnology 224, Colloque INSERM, John Libbey Eurotext Ltd. (1992), p. 195.
Cody, S.H., Glynn, M.K., Farrar, J.A., Cairns, K.L., Griffin, P.M. J. Kobayashi, J. et al., (1999) An outbreak of Escherichia coli O157:H7 infection from unpasteurized commercial apple juice, Annals of Internal Medicine 130 (3), pp. 202–209.
Farkas, D. F., & Hoover, D. G. (2000). High pressure processing. Journal of Food Science, 65, pp. 47-64 (Article).
Hauben, K.J.A., Wuytac, E.Y., Soontjens, C.F., and Michiels, C.W. (1996) High-pressure transient sensitization of Escherichia coli O157:H7 to lysozyme and nisin by disruption of outer membrane permeability. Journal of Food Protection 59, pp. 350–359.
Heinz, V. & Knorr, D. (2002). Effects of High Pressure on Spores Ultra High Pressure Treatments of Foods (edited by M.E.G. Hendrickx, D. Knorr, L. Ludikhuyze, A. Loey & V. Heinz). Pp. 77–113. New York: Springer USA.
Hendrickx, M., Ludikhuyze, L., Van den Broeck, I., & Weemaes, C. (1998). Effects of high pressure on enzymes related to food quality. Trends in Food Science & Technology, 9(5), pp. 197-203 (Article).
Heremans, K. (2003) The effect of high pressure on biomaterials. In: Ultra High Pressure Treatments of Foods ed. Marc E.G. Hendrickx and Dietrich Knorr. pp. 23-52. Kluwer Academic/ Plenum Publishers, New York.
Hite, B.H. (1899). The effect of pressure in the preservation of milk. Bulletin (West Virginia University. Agricultural Experiment Station), 58, pp. 15–35
Hite, B. H., Giddings, N. J., &Weakley, C.W. (1914). The effect of pressure on certain microorganisms encountered in the preservation of fruits and vegetables. Bull. of the West Virginia Agricultural Experimental Station, 146, pp. 1–67
Hogan, E., Kelly, A.L. Sun, D.-W. (2005) 1-High Pressure Processing of Foods: An Overview. In: Emerging Technologies of Food Processing. Academic Press, pp. 3-32 (Article)
Ibarz, A., Gonzalez, C., Barbosa-Canovas, G.V. (2004) Kinteic models for water adsorption and cooking time in chickpea soaked and treated by high pressure. J. Food Eng., 63(4) pp. 467-472 (Article)
Isaacs, N.S. and Chilton, P. (1995) Microbial inactivation mechanisms. In: D.A. Ledward, D.E. Johnston, R.G. Earnshaw and A.P.M. Hastings, Editors, High pressure processing of foods, Nottingham University Press, Nottingham, UK, pp. 65–179.
Kalchayanand, N., Sikes, T., Dunne, C.P., Ray, B. (1994) Hydrostatic pressure and electroporation have increased bactericidal efficiency in combination with bacteriocins. Appl. Environ. Microbiol. 60(11) pp. 4174–7.
Kalchayanand, N., Sikes, T., Dunne, C.P., Ray, B. (1994) Hydrostatic pressure and electroporation have increased bactericidal efficiency in combination with bacteriocins. Appl Environ Microbiol 60(11) pp. 4174–7.
Kalchayanand, N., Sikes, A., Dunne, C.P., Ray, B. (1998a) Factors influencing death and injury of foodborne pathogens by hydrostatic pressure-pasteurization. Food Microbiol. 15 pp. 207–214.
Kalchayanand, N., Sikes, A., Dunne, C.P., Ray, B. (1998b) Interaction of hydrostatic pressure, time and temperature of pressurization and pediocin AcH on inactivation of foodborne bacteria. Journal of Food Protection 61 (1998), pp. 425–431.
Koutchma, T. (2014a). Fundamentals of HPP Technology/ In-Container HPP Principle; Adapting High Hydrostatic Pressure (HHP) for Food Processing Operations; pp.5. Guelph, ON, Canada: Agriculture and Agri-Food Canada, Guelph Food Research Center
Li, X., Mao, L., He, X., Ma, P., Gao, Y., Yuan, F. (2018) Characterization of β-lactoglobulin gels induced by high pressure processing. Innov. Food Sci., Emerg. Technol. 47 pp. 335-345 (Article)
Linton, M., McClements, J.M.J. and Patterson, M.F. (1999) Inactivation of Escherichia coli O157:H7 in orange juice using a combination of high pressure and mild heat. Journal of Food Protection 62, pp. 277–279.
McCarthy, M. (1996) E. coli O157:H7 outbreak in USA traced to apple juice, Lancet 348 p. 1299.
Mor-Mus, M., Escriu, R., Yuste, J. (2014) Chapt. 5 – Microbiological aspects of high-Pressure Processing. In: Emerging Technologies of Food Processing (2nd edt.). Academic Press, pp. 77-90 (Article)
Muntean, M.-V., Marian, O., Barbieru, V., Catunescu, G.M. Ranta, O., Drocas, I. Terhes, S. (2016) High Pressure Processing in Food Industry – Characteristics and Applications. Proc. 5th Int. Conf. ‘Agriculture for Life, Life for Agriculture’ Agric. Agric. Sci. Procedia 10 pp. 377-383 (Article)
Pandrangi, S., Balasubramanian, V.M., Tao, Y., Sun, D-W. (2014) Chapter 2 – High Pressure Processing of Salads and Ready Meals. In: Emerging Technologies of Food Processing (2nd edt.). Academic Press, pp. 25-34 https://doi.org/10.1016/B978-0-12-411479-1.00002-4
Patras, A., Brunton, N. P., Da Pieve, S., & Butler, F. (2009). Impact of high pressure processing on total antioxidant activity, phenolic, ascorbic acid, anthocyanin content and colour of strawberry and blackberry purées. Innovative Food Science & Emerging Technologies, 10(3), pp. 308-313.
Patterson, M.F., Quinn, M., Simpson, R., and Gilmour, A. (1995) Sensitivity of vegetative pathogens to high hydrostatic pressure treatment in phosphate-buffered saline and foods. Journal of Food Protection 58, pp. 524–529.
Pyatkovskyy, T.I., Shynkaryk, M.V., Mohamed, H.M. Yousef, A.E., Sastry, S.K. (2018) Effects of combined high pressure (HPP), pulsed electric field (PEF) and sonication treatments on inactivation of Listeria innocua. J. Food Eng. 233 September pp. 49-56 (Article)
Rasanayagam, V., Balasubramaniam, V.M., Ting, E., Sizer, C.E., Bush, C., and Anderson, C. (2003) Compression heating of selected fatty food materials during high-pressure processing. Journal of Food Science, 68(1) pp. 254–259.
Rastogi, N.K., and Niranjan, K. 1998. Enhanced mass transfer during osmotic dehydration of high-pressure treated pineapple. Journal of Food Science, 63(3) pp. 508–511.
Rastogi, N.K., Angersbach, A., and Knorr, D. (2000a) Combined effect of high hydrostatic pressure pretreatment and osmotic stress on mass transfer during osmotic dehydration, Proceedings of 8th International Congress of Food and Engineering (ICEF’8) held at Mexico. April 9–13, 2000
Rastogi, N.K., Angersbach, A., Niranjan, K., and Knorr, D. (2000b) Rehydration kinetics of high pressure treated and osmotically dehydrated pineapple. Journal of Food Science, 65(5), pp. 838–841
Rastogi, N.K., Angersbach, A., and Knorr, D. 2000c. Synergistic effect of high hydrostatic pressure pretreatment and osmotic stress on mass transfer during osmotic dehydration. Journal of Food Engineering, 45(1) pp. 25–31.
Rastogi, N.K., Angersbach, A., and Knorr, D. 2003. Combined effect of high hydrostatic pressure pretreatment and osmotic stress on mass transfer during osmotic dehydration, In Transport Phenomena in Food Processing (ed. J. Welti-Chanes), CRC Press, USA. pp 109–121
Rastogi, N. K., Raghavarao, K. S. M. S., Balasubramaniam, V. M., Niranjan, K., & Knorr, D. (2007). Opportunities and challenges in high pressure processing of foods. Critical Reviews in Food Science and Nutrition, 47(1), pp. 69-112 (Article)
Smelt, J.P.M. (1998) Recent advances in the microbiology of high pressure processing. Trends in Food Science & Technology 9, pp. 152–158
Smith, K., Mendona, A., Jung, S. (2009) Impact of high-pressure processing on microbial shelf-life and protein stability of refrigerated soymilk. Food Microb. 26(8) pp. 794-800 (Article)
Teo, A.Y.L., Ravishankar, S. and Sizer, C.E. (2001) Effect of temperature, high-pressure treatment on the survival of Escherichia coli O157:H7 and Salmonella in unpasteurized fruit juices, Journal of Food Protection 64 (8), pp. 1122–1127.
Tintchev, F., Kuhlmann, U., Wackerbarth, H. et al. (2009). Redox processes in pressurised smoked salmon studied by resonance Raman spectroscopy. Food Chemistry, 112, pp. 482–486 (Article).
Tintchev, F., Wackerbarth, H., Kuhlmann, U. et al. (2010). Molecular effects of high‐pressure processing on food studied by resonance Raman. Annals of the New York Academy of Sciences, 1189, pp. 34–42 (Article)
Toepfl, S., Mathys, A., Heinz, V. & Knorr, D. (2006). Review: potential of high hydrostatic pressure and pulsed electric fields for energy efficient and environmentally friendly food processing. Food Reviews International, 22, pp. 405–423 (Article).
Torres, J.A. & Velazquez, G. (2005) Commercial opportunities and research challenges in the high pressure processing of foods. J. Food Eng. 67 (1-2) pp. 95-112 (Article)
Tsai, M.-J., Cheng, M.-C., Chen, B.-Y., Wang, C-Y. (2018) Effect of high-pressure processing on immunoreactivity, microbial and physicochemical properties of hazelnut milk. Int. J. Food Sci. Technol. 53(7) pp. 1672-1680 (Article)
Wilson, D.R., Dabrowski, L., Stringer, S., Moezelaar, R. & Brocklehurst, T.F. (2008). High pressure in combination with elevated temperature as a method for the sterilisation of food. Trends in Food Science and Technology 19, pp. 289–299
Yang, Y., Xia, Y., Wang, G., Tao, L., Yu, J., & Ai, L. (2019). Effects of boiling, ultra-high temperature and high hydrostatic pressure on free amino acids, flavor characteristics and sensory profiles in Chinese rice wine. Food Chemistry, 275, pp. 407-416 (Article).
Yen, G. C., & Lin, H. T. (1996). Comparison of high pressure treatment and thermal pasteurization effects on the quality and shelf life of guava puree. International Journal of Food Science & Technology, 31(2), pp. 205-213 (Article)
2016). Effects of high hydrostatic pressure processing and subsequent storage on phenolic contents and antioxidant activity in fruit and vegetable products. International Journal of Food Science and Technology, 52, pp. 3–12 (Article) , & (
Revisions: New material added including references and updates on current thinking about HPP.
Hope you don’t mind as I used a good part of this article for one of my engineering essays. You should make it a cornerstone article !