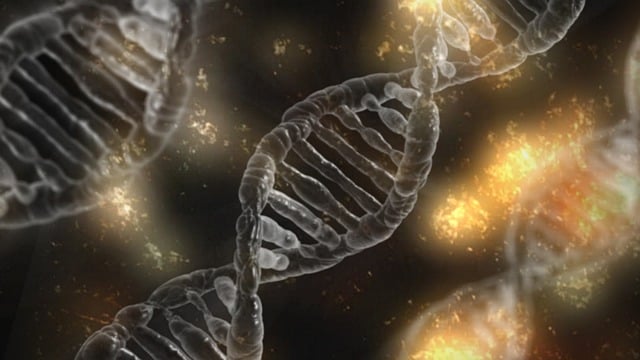
DNA (Deoxyribonucleic acid) is a molecule which is fundamental to life. For a start it is the code that determines the structure and nature of all cells and ultimately all life as we know it.
The History Behind Discovery And Function Of Deoxyribonucleic Acid
In the early days of biochemistry, many scientists thought that proteins were responsible for containing all the hereditary material. They were more complex than DNA even though they knew of its existence.
For a start all proteins contain 20 different amino acids which form polypeptide chains. There are many more combinations of amino acids than could be feasible with the units that make up DNA.
The first study which started to reveal that DNA was hereditary material came with the scientist Fred Griffith. He was working on two strains of Pneumoccocus bacteria. One was virulent S bacteria and the other was nonvirulent R bacteria. He took the DNA from heat-killed virulent S strains and added it to the nonvirulent R strain where it became virulent as a result. On that basis it was reasoned that DNA probably was the chemical responsible for hereditary – it was the genetic material of cells. More proof was needed however!
Another type of material extracted from cells were the chromosomes. These are composed of both DNA and protein. They change during cell division and replication.
Two scientists, Hershey & Chase did experiments on bacteriophages which are viruses that attack bacteria. In a very simple experiment they wanted to know what the bacteriophage was using to replicate itself in a bacteria. They labelled the DNA with radioactive phosphorous i.e. 32P whilst the protein was labelled with radioactive 32S. It was the radioactive phosphorous which was injected into the bacteria and provided extremely strong evidence that DNA was actually the material holding the genetic code.
DNA Structure
DNA structure was one of those stories of biochemistry which has proved fascinating to many. DNA contains four bases, A, T, C and G which are nucleotides. They are:-
- adenine (A)
- thymine (T)
- cytosine (C)
- guanine (G)
Erwin Chargaff worked out the amounts of each base of DNA in a somatic or body cell. The amounts were 30.3% each of adenine and thymine, and 19.5% of guanine and 19.9% cytosine.
Based on these amounts and ratios, we have Chargaff’s Rule which shows that adenine must be paired with thymine. Guanine then has to be paired with cytosine.
These bases are bound to each other with very weak hydrogen bonds.
In the 1950’s, Rosalind Franklin took X-ray diffraction pictures of DNA crystals. From these, the scientists, Watson & Crick built the first model of DNA. Based on their research they were honoured with a Nobel Prize.
Their DNA model has two strands coiled around each other in a double helix. The backbone to which the bases are connected to are made up of the pentose sugar deoxyribose which is bonded to a phosphate group that bridges the next pentose sugar. Each pentose sugar has a base attached.
The phosphate bridge is known as a phosphodiester bond.
In the centre of the helix are the nitrogen bases which are weakly bonded together using hydrogen bonds. The DNA double helix can thus be thought of as a ladder. The rungs are the nitrogenous bases and the sides or legs are the sugar plus phosphate backbone.
The Helix Structure
The helix structure is right-handed. It has a right-hand twist. One turn is made up of 10 base pairs.
Left twisted DNA is known as Z-DNA or southpaw DNA.
Mutations called hotspots occur when right and left twisted DNA meet.
On a more chemical basis, DNA (deoxyribonucleic acid) is made up of subunits called nucleotides. All nucleotides are composed of:-
- 5-carbon sugar
- nitrogenous base
- phosphate group
One strand of DNA goes from the 5′ to the 3′ end based on the chemical structure of the sugars. The complementary strand i.e. the other strand must run in the opposite direction going 3′ to 5′ in terms of its sugars. They are described as antiparallel strands.
The nitrogenous bases are purines which are double ring structures – they are adenine (A) and guanine (G). The single ring structures thymine (T) and cytosine (C) are pyrimidines.
It appears then that purines can only base pair with pyrimidines.
To base pair guanine with cytosine requires three hydrogen bonds. To base pair adenine with thymine requires two hydrogen bonds.
Differences Between DNA and RNA
RNA (ribonucleic acid) is similar to deoxyribonucleic acid but the thymine (T) is replaced with uracil (U). The main reasons are that uracil is less stable than thymine. DNA has evolved to be as robust as possible because it is a long-term storage molecule of genetic information which should be as ‘incorruptible’ as possible. RNA however is relatively short lived for all the activities it is needed for so the presence of uracil is less of an issue. The presence of uracil also means that DNA polymerases which are needed for the repair of DNA as well as replication can straightforwardly distinguish between the two types of ribonucleic acid.
The Industrial Production Of DNA
Producing DNA on an industrial scale involves advanced biotechnological methods. The DNA is primarily used in fields like medicine, agriculture, research, and industrial biotechnology.
- Plasmid DNA Production (Most Common Method)
- Host Organism: DNA is typically produced using genetically engineered Escherichia coli (E. coli) bacteria, which are easy to grow and manipulate.
- Fermentation: Large-scale fermentation tanks are used to grow the bacteria under controlled conditions, optimizing temperature, pH, and nutrients.
- Plasmid Isolation: Plasmids (circular DNA molecules) are extracted from bacterial cells after fermentation.
- Steps: The cells are lysed (broken open) chemically or mechanically, and the plasmid DNA is purified using methods like ultracentrifugation or column chromatography.
- Synthetic DNA Production
- Chemical Synthesis: Short DNA fragments, called oligonucleotides, are chemically synthesized using automated synthesizers. This method is suited for producing specific sequences of DNA for primers, probes, or gene synthesis.
- Enzymatic Assembly: Long DNA strands can be assembled by stitching together oligonucleotides using DNA polymerase or ligase enzymes.
- PCR-Based Amplification
- DNA sequences are amplified using the Polymerase Chain Reaction (PCR) in reactors. This method is often used for medium-scale production of specific sequences.
- Cell-Free Systems
- Cell-free systems use engineered enzymes to replicate DNA without using living cells. While not yet as widespread as plasmid-based methods, this is an emerging technology with potential for faster and cleaner DNA production.
- Quality Control
- After production, DNA is tested for purity, integrity, and sequence accuracy using techniques like spectrophotometry, gel electrophoresis, and next-generation sequencing (NGS).
Uses of Industrial Deoxyribonucleic Acid
- Therapeutic Applications
- Gene Therapy: Plasmid DNA is used as a vector to deliver therapeutic genes into cells.
- DNA Vaccines: DNA-based vaccines, such as some COVID-19 vaccines, introduce genetic material encoding antigens to stimulate an immune response.
- CAR-T Therapy: DNA sequences are used to engineer T-cells to target specific cancers.
- Diagnostics and Research
- Genetic Testing: DNA probes and primers are produced for identifying genetic mutations or diseases.
- Molecular Biology Research: DNA is a crucial tool for cloning, sequencing, and studying gene functions.
- Synthetic Biology
- Gene Editing: Tools like CRISPR-Cas9 rely on synthetic DNA for guide RNAs and repair templates.
- Customized Organisms: DNA is used to engineer microbes for producing biofuels, pharmaceuticals, or specialty chemicals.
- Agriculture
- Genetically Modified Organisms (GMOs): DNA is used to create crops with enhanced traits like pest resistance or drought tolerance.
- Synthetic Seeds: DNA can be used to develop new plant varieties with improved genetics.
- Industrial Biotechnology
- Enzyme Production: DNA encoding industrial enzymes is used to engineer microbes for large-scale enzyme production.
- Biomanufacturing: DNA is used to engineer microorganisms for producing bioplastics, biofuels, or high-value compounds.
- Forensics and Biobanking
- DNA is used in forensic science for criminal investigations or in biobanking for preserving genetic material.
Scale of Industrial DNA Production
- Industrial DNA facilities operate at both small (micrograms) and large (kilograms) scales, depending on the application. DNA vaccines, for instance, require kilograms of DNA, while genetic research or diagnostics may only need milligrams.
DNA (deoxyribonucleic acid) is probably the most important molecule in any biological system. Simply put, it is the store or repository of genetic information. Without it, cells would be unable to replicate themselves or to adapt to the environment. With advances in biotechnology, the demand for high-quality, scalable DNA production continues to grow, especially in precision medicine and synthetic biology.
Nice and simple and not wrong!